DBP3: Multiscale Modeling of DAT Function in Dopamine (DA) Neurons
DBP3: Multiscale Modeling of DAT Function in Dopamine (DA) Neurons
A. Collaborating Investigators: Alexander Sorkin,1 Simon Watkins,1 Ethan R. Block,2 GonzaloTorres,3 Ivet Bahar,1 James Faeder,1 Robert Murphy,4 Terry Sejnowski,5 Tom Bartol4
B. Institutions: 1Pitt, 2Chatham U, 3U of Florida, 4Carnegie Mellon U, 5Salk
C. Funding Status: R01DA14204(Sorkin) 5/1/01-4/7/17; R01DA038598 (Torres); 9/15/14 - 6/30/19
D. Driving relationship between DBP3 and TR&Ds: Two important advances in the past funding term now open the way to multiscale structure-based, spatiotemporally realistic simulations of DA reuptake by DA neurons. The first is the structural resolution of Drosophila melanogaster DA transporter, dDAT,46 and its interactions with antidepressants47 and psychostimulants,48 which permitted us (TR&D1) to elucidate the structural dynamics of human DAT (hDAT),49 as well as the molecular mechanism of its interactions with cocaine and AMPH.50 The second breakthrough is the quantitative characterization of the spatiotemporal distribution of DAT in the striatum by the Sorkin lab. This was enabled by novel immunofluorescence labeling methods with transgenic knock-in mice that express hemagglutinin (HA) epitope-tagged DATs.51 The data was essential to building a first MCell model (Fig VII.3) which indicated that the geometry near the active zone and the spatial distribution of DATs significantly affect the effectiveness of DA reuptake. Data obtained by others52,53 also suggest that in the striatum firing patterns alter the efficiency of DA receptor activation. Further data will be supplied by Pitt and U of Florida collaborators for the effect of psychostimulants/drugs on DA transport. Our goal is to construct the first spatiotemporally realistic model of DA reuptake and examine its dynamics in response to different types of firing patterns and in the presence of different drugs or psychostimulants.
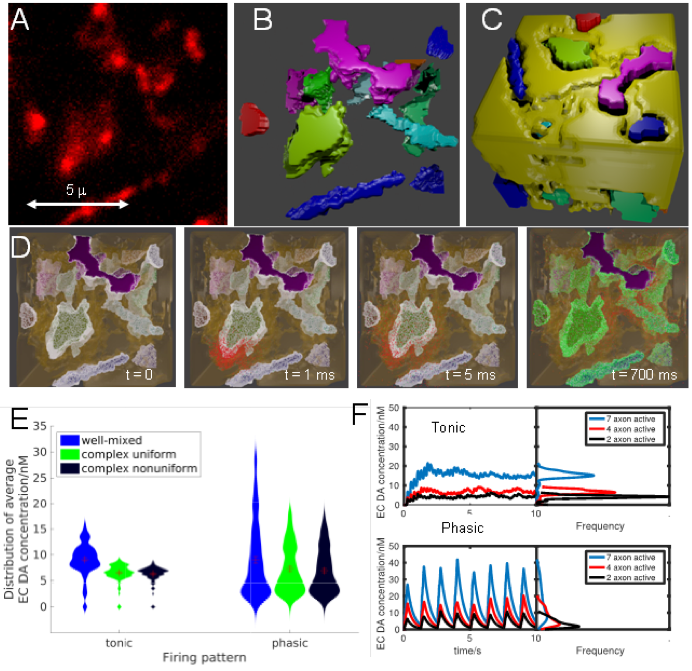
Fig VII.3. Modeling and simulation of dopamine (DA) uptake by DA neurons.
A. Distribution of HA-DAT in mouse brain striatum. Slices were labeled with DAT-Nt antibodies, and conjugated with Cy3 (HA, red). The fluorescence image consists here of 18 slices with 0.4 μm. B. 3D reconstruction of A, visualized with Blender. Different colors shows different axonal segments. C. Isometric view of the simulation box. The remaining portions are filled with cells that do not express DAT. D. Snapshots from MCell simulations. Color code: red, DA; white, outwards facing DAT; green, inward-facing DAT. E. Distribution of average extracellular (EC) DA level from runs with different settings: blue, well-mixed model; green, realistic geometry with uniform distribution of DAT; and black, realistic geometry with heterogeneous distribution of DAT derived from fluorescence images. Results are shown for 2 different firing patterns, tonic and phasic, both with same average frequency. F. Time evolution of EC DA concentration for tonic (top) and phasic (bottom) firing, for different numbers of active axonal termini (right) and their histograms (left).
E. Innovation: This will be the first development of a spatially realistic model for DA axonal arbors and DAT localization near active zones, which will provide a framework for simulating DA reuptake using as input time-resolved super-resolution image data with resolution of 120 nm (lateral) x 240 nm (axial). This, combined with advances in BTRR technology will permit a first molecular-to-cellular structure-based study of DA signaling and its modulation by psychostimulants at the time scale of milliseconds to minutes.
F. Methods and Procedure: Aim 1. In silico reconstruction of DA neurons morphology and molecular distributions. The Sorkin lab will provide stacks of images obtained with the technology described recently,54 which includes immuno-histochemical analysis of brain slices, confocal fluorescence microscopy, EM and immunogold-silver labeling of DAT with transgenic HA knock-in mouse brain. These will drive the development and implementation of (i) high-quality image registration and segmentation methods and (ii) a geometry preparation pipeline for MCell/CellBlender, by TR&D2 and TR&D4. Thus far, we have used a semi-automated 3D reconstruction method55 to build a simulation box of 772 m3 containing 16 axon terminals (Fig VII.3 A-C). The spacing between DA neurons (void fraction: 0.298) and the spatial distribution of DATs (220,000 of them) were deduced from EM images. Using newly developed technologies, TR&D2 & 4 will reconstruct with high- fidelity the full data (382 micrographs for 9000–17,000 m2 striatum tissue) with/without AMPH.56
Aim 2. Development of a kinetic model for DA/psychostimulant binding/unbinding and DAT alternating access mechanics. This aim will drive the development of the new hybrid methodology that combines coMD28,57 and WE58,59 for generating molecular data on the populations of substates and their rates of transitions (TR&D1 aims 1 and 3) . These will serve as input for MCell. They will be benchmarked and iteratively refined using electrophysiology and kinetics data from both DBP1 and DBP3 experiments and the literature, in addition to earlier applicable results from expensive full-atomic studies.50,60 We already studied the structural dynamics of hDAT61 and its structural homologs,62-67 and the effects of psychostimulants on DAT dynamics.50 These will be used in the hybrid coMD-WE methodology (TR&D1 aim 3) that will be iteratively refined using DAT transport cycle data as benchmark. We will perform free energy perturbation (FEP) and adaptive biasing force (ABF) calculations, and assess ligand selectivity (see e.g.68), and current-voltage relations.
Aim 3. MCell simulations of DA reuptake events under different conditions, and trajectory analysis. This will require enhancement of the simulation and interfacing capabilities of MCell (TR&D2 aims 1 and 3) in collaboration with TR&D3 (e.g. spatially structured, multi-state, multi-component molecules). Vesicular DA discharge will be modeled as quantal release from active zones. A distance threshold of 20 Å will be adopted for DA-DAT recognition, consistent that observed in our simulations for DAT or LeuT.50,69-72 Our preliminary runs show that (i) DA molecules diffuse away from the active zone within ~5 ms (Fig VII.3D), (ii) axonal geometry and heterogeneous distribution of DATs significantly affect the distribution of DA levels in the striatal EC region (Fig VII.3E), underscoring the importance of doing MCell simulations; and (iii) the propensity to reach the EC levels (of 10 nM) required for D2R activation is much higher in the presence of phasic release of DA, as opposed to tonic (Fig VII.3F). The variance is greatly reduced when a fraction of striatal axons are rendered inactive (e.g. PD model). We will establish the conditions under which normal DA transmission is ensured, and study how drugs alter the dynamics. Image and electrophysiology data from our collaborators73-75 will serve as test bed for validating our results. Cocaine blocks the DA binding site,50,76-78 resulting in increased EC [DA]; we will examine under which conditions these levels approach that (~1mM)79 required for activating low affinity DA receptors. Data from fast scan cyclic voltammetry experiments in the presence of AMPH and L-DOPA80 will further help test the predictions. Data from DBP1 will further help elucidate AMPH-stimulated events.
Pitfalls and alternative strategies. The time scale of occupancy of binding site on DAT by cocaine (hours)81 is longer compared to the timescales (seconds to minutes) of DA signaling. We may need long simulations in order to obtain statistically significant data. Alternatively, simulations with mixed (explicit and implicit) interactions will be considered. Similar strategies may be required for PD models where the DA neuronal activity is slowed down,82 or for long-time exposures (>15 min) to AMPH or L-DOPA.
DBP2: Regulation of binding to PSD-95 and its relation to AMPA receptor trafficking
DBP2: Regulation of binding to PSD-95 and its relation to AMPA receptor trafficking
A. Collaborating Investigators: Mary Kennedy,1 Terry Sejnowski,2 Tom Bartol,2 I. Bahar,3 J Faeder3
B. Institutions: 1Caltech, 2Salk, 3Pitt
C. Funding Status of Project: Caltech Institutional Funds
D. Driving relationship between TR&D2 and DBP2: Electrophysiologically observed changes in synaptic strength that follow Ca2+ influx can have a variety of underlying biochemical mechanisms, and usually involve the concerted action of several partially redundant regulatory mechanisms.33,34 It is essential to fully define the biochemical mechanisms that produce long-term potentiation (LTP) and long-term depression (LTD) in order to fully understand brain function. This DBP will test and explore one possible underlying mechanism for regulation of the number of AMPA receptors (AMPARs) that are stabilized at the postsynaptic site. A well-supported hypothesis states that a 3-step mechanism is involved in the initial recruitment of AMPARs to cortical and hippocampal excitatory synapses: “exocytosis at extra/perisynaptic sites, lateral diffusion to the synapse, and a subsequent rate-limiting diffusional trapping”.35 Steps 1 and 3 are tightly regulated by synaptic activity. We now have a reasonably good idea about the particular proteins that contribute to the regulation of these steps, but we don’t yet understand the precise dynamics that organize these steps into a well-regulated system that integrates the functions of synapses, neurons, and the brain.
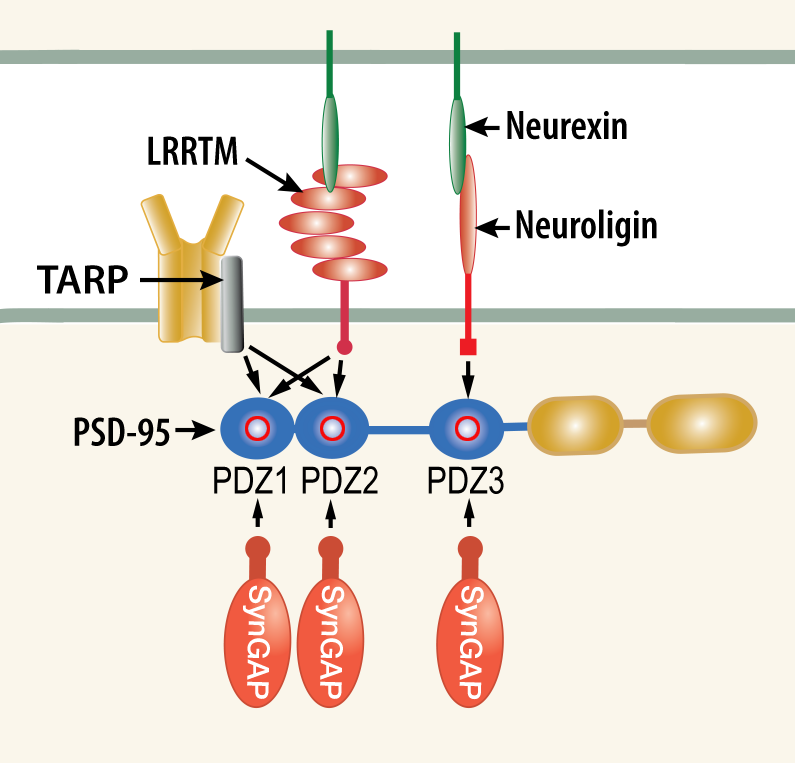
Fig VII.2. Schematic representation of neuroligin, SynGAP, LRTTM and TARP, that bind to PSD-95 PDZ domains, and regulate AMPAR stabilization near the PSD.
Specifically, this DBP tests the hypothesis that trapping of AMPARs in the postsynaptic density (PSD) is regulated by competition for binding to the PDZ domains of the postsynaptic scaffold protein PSD-95. We will employ MCell36 and two sets of experiments to study how competition among four key PSD proteins plays a critical role in determining the number of AMPARs at a postsynaptic site. The four proteins are neuroligin (NLG1),37 transmembrane AMPAR-regulatory protein (TARP),38,39 leucine-rich-repeat transmembrane protein (LRRTM),40 and synaptic GTPase activating protein (synGAP)41,42 (Fig VII.2). Two of these, LRRTM and TARP, bind to AMPAR and are believed to participate in trapping of AMPARs in the PSD. The objectives also test the hypothesis, based on recent findings in the Kennedy lab, that the phosphorylation of synGAP by Ca2+/CaMKII alters the balance of competition among these proteins (see Fig I.3 in the Overall Section).43 A central consequence is that modulating the binding affinity of these proteins to PDZ domains contributes to activity-dependent changes in synaptic strength.
E. Resulting Innovations: This project will drive development of the spatial extensions to BioNetGen Language (BNGL)44 for MCell in TR&D2 in two ways: (i) the competitive interactions among the molecules for the PDZ domains results in a reaction mechanism with a high degree of combinatorial complexity and (ii) the trans-synaptic complexes between neurexin and NLG1 and LRRTMs45 (Fig VII.2) will be represented as structures that bridge between pre- and postsynaptic cells.
F. Methods and Procedures: Aim 1: We will measure the affinities of the carboxyl terminal tails of NLG1, TARP-ɣ8, and LRRTM2 for the three PDZ domains of PSD-95 by BIAcore surface plasmon resonance (SPR) methods. This objective will provide necessary parameters for the model proposed in Aim 2. Affinities for binding of synGAP to PDZ domains, before and after phosphorylation of synGAP by CaMKII, have already been measured in the Kennedy lab. Thus, methods are in place to carry out this objective. The interaction of PDZ domains with AMPAR, NLG1 and SynGAP C-terminal motif QTRV, as well as the neuroligin-neurexin interactions will be analyzed using the methods developed in TR&D1, toward characterizing the molecular basis of the competition of these proteins for binding to PSD-95.
Aim 2: We will use MCell to simulate diffusion of NLG1, TARPs, and LRRTMs in the membrane of postsynaptic spines, and their interactions with PSD-95 immobilized near the membrane within the PSD. Prior evidence indicates that synGAP may be immobilized in the PSD by associations in addition to its binding to the PDZ domains of PSD-95. Therefore, we will simulate diffusion of synGAP and its dynamic association with immobilized PSD-95 under three conditions; diffusing freely in the cytosol, tethered to the spine membrane, and tethered to the membrane within the volume of the PSD. We will assess the dynamics of the competition of these proteins for binding to PDZ domains of PSD-95. For example, we will measure the equilibrium mixtures of the four proteins in the PSD before and after phosphorylation of synGAP (which reduces its affinity for synGAP ~10 fold.) We will use the experimental measurements of binding affinities already obtained for synGAP and to be gathered in Aim 1, as parameters in the simulations. We will include in the simulations binding of LRRTMs and NLGs to presynaptic neurexins across the synaptic cleft (the affinity of which has been measured by other labs). One goal will be to establish a proof of principle that competition for binding to PDZ domains of PSD-95 is physiologically significant given the range of experimentally determined parameters and numbers of proteins. A second will be to measure how changes in the affinities for PDZ domains of PSD-95, for example reduced affinity of phosphorylated synGAP, can alter the complement of proteins immobilized in the PSD.
Aim 3: We will carry out experiments to measure changes in the ratios of PSD-95 to synGAP, NLG1, LRRTM1/2, and TARP-ɣ8 in PSDs and in PSD-95 complexes isolated from neuronal cultures before and after induction of chemLTP, a pharmacological treatment that mimics physiological induction of LTP. The amounts of the proteins will be measured by quantitative immunoblotting with specific antibodies. We will test the hypothesis that synGAP plays a central role in regulation of the composition of the PSD-95 complex by comparing these measured ratios among neurons cultured from synGAP homozygotes, synGAP heterozygotes, and wild type litter mates. These experiments will also be used as a first test of whether results of the simulations proposed in Aim 2 reflect an observable physiological process.
DBP1: Dynamics of Neurotransmitter Transporters: Molecular and Cellular Interactions
DBP1: Dynamics of Neurotransmitter Transporters: Molecular and Cellular Interactions
A. Collaborating Investigators: Susan G Amara,1 Gonzalo Torres,2 Delany Torres-Salazar,1 Jennie Garcia-Olivares,1 Ivet Bahar,3 Mary Cheng 3 Bing Liu,3 James Faeder,3 Terry Sejnowski,4 Tom Bartol4
B. Institutions: 1NIH, 2University of Florida, 3Pitt and 4Salk
C. Funding Status of Project: 1ZIAMH002946-03 (Amara); R01 DA038598 (Torres) 9/15/14 - 6/30/19
D. Driving relationship between DBP1 and TR&Ds. Sodium-coupled neurotransmitter (NT) transporters regulate neurosignaling in the CNS and prevent neurotoxicity by clearing excess NT from the synapse. Significant studies have been made in recent years for elucidating their mechanisms of function, including the contributions of TR&D1 and DBP1 during the past term to characterizing (i) the structure-encoded dynamics of excitatory amino acid transporters (EAAT1-3) and dopamine transporter (DAT) or its structural homolog LeuT and (ii) the molecular basis of their cellular function/dysfunction, Yet, several aspects of NT transport remain unknown. First, many transporters also act as anion (e.g. chloride, Cl-) channel. Anion conductance has been suggested to promote electrogenic Glu- uptake and serve as a sensor for regulating the release of additional Glu-. The Amara lab and others found that Cl- channeling is structurally coupled to Glu- transport, but the molecular mechanism of coupling is unclear. Second, NT transporters also allow for the efflux (or reverse transport) of NT, modulated by regulatory proteins such as G protein β-γ subunits (Gbg) and CaMKII, addictive drugs such as cocaine and amphetamine (AMPH), or lipids. How the transporter structure adapts to enable NT efflux remains to be understood. Third, recent studies by the Amara lab highlight the key role of intracellular (IC) AMPH in modulating the function and internalization of transporters; it is not clear how protein-protein interactions (PPIs) stimulated by AMPH alter or impair neurosignaling. This DBP aims at shedding light on these fundamental questions.
E. Innovation: We will develop (i) an advanced protocol that will permit users to combine ENMs, aMD, and co-MD27,28 to explore substrate/ion permeation paths; (ii) automated methods for evaluating disulfide trapping and solvent/reagent accessibility experiments; (iii) the first comprehensive quantitative network model for AMPH-stimulated signaling in DA neurons. Combined experimental and computational results will provide a mechanistic understanding of the orchestration of IC AMPH-triggered PPIs and an in silico platform for developing new hypotheses for controlling AMPH actions or regulating neurotransmission.
F. Methods and Procedure: Aim 1. Test the hypothesis that Cl-/anion channeling by EAAT1 involves a structural change into an intermediate state and identify the location of Cl- permeation pore and key residues lining the pore. Using the SCAM method, the Amara lab heterologously expressed in oocytes, single Cys substitutions at EAAT1 residues predicted by the Bahar lab (M89C, counterpart of Gltph V51) to be within the anion permeation pathway. In at least 3 of the substitutions we observed an approximately 50% reduction in the macroscopic current amplitude after application of MTSES, a negatively charged sulfhydryl-reactive reagent. We observed comparable results with MTSACE, a similar, but non-charged reagent, ruling out that the observed current reductions were caused by electrostatic interactions. Moreover, our results consistently showed that substrate translocation is not altered by these modifications (Fig VII.1). Using a combination of molecular modeling, substituted cysteine accessibility, electrophysiology and glutamate uptake assays, we identified a chloride-channeling conformer, iChS, transiently accessible as EAAT1 reconfigures from substrate/ion-loaded into a substrate-releasing conformer. Opening of the anion permeation path in this iChS is controlled by the elevator-like movement of the substrate-binding core, along with its wall that simultaneously lines the anion permeation path (global); and repacking of a cluster of hydrophobic residues near the extracellular vestibule (local).1
Fig VII.1. Application of 10 mM MTSES reduced anion current amplitudes but not substrate transport. (A) Time dependence of MTSES modification of anion (NO3-) macroscopic current amplitudes measured at +60 mV in oocytes expressing the mutant M89C (τ= 5.2 ± 0.2 seconds; n=5). Black bar indicates the duration of the application. (B) Current-voltage relationship of the same cells represented in A before (red circles) and after (cyan triangles) application of MTSES, and after application of 1mM DTT (green diamonds) following the application of MTSES. (C) Bar representation of the current amplitudes at +60 mV from B. (D) Current-voltage relationship representing transport currents before (red diamonds) and after (cyan diamonds) the application of MTSES (currents were measured in chloride-based solution; n=5). (E) Bar representation of the currents at -120 mV from D. (F) radiolabeled Glu- uptake before and after application of MTSES remains unchanged (n = 34).
Aim 2. Test the hypotheses that G-βγ binding to DAT drives an allosteric conformational change in DAT, which in turn, promotes DA efflux. We generated a first structural model for DAT-Gβγ complex and identified two interfacial salt bridges between Gβγ and hDAT C-terminus. DA efflux assays by Torres using the mutants R588A and R610A showed preliminary results in support of our model. Our simulations of hDAT-Gbg complex in explicit water and lipid revealed a water channel, which is to our knowledge the first such observation in hDAT. This may be indicative of a possible anion channel, or DA efflux path. Notably, highly conserved D79 and D421 line the DA translocation path; whereas E117 and E491 line this new water channel. We will establish the role of this channel by iterative, coordinated computations including metadynamics to quantify selective ion permeation and experiments.
Aim 3. Model and analyze the dynamics of the PPI network that mediates AMPH-stimulated DAT internalization and other signaling events in DA neurons. New data from the Amara lab, along with those from the literature and models determined in TR&D1, will be used to build a comprehensible BioNetGen model for Rho- and PKA-dependent AMPH actions, which will be iteratively tested and validated against time-resolved concentration and activity data to be supplied by the Amara lab.
- Cheng MH, Torres-Salazar D, Gonzalez-Suarez AD, Amara SG, Bahar I. (2017) Substrate transport and anion permeation proceed through distinct pathways in glutamate transporters. ELife 6: pii: e25850
Driving Biomedical Projects
MMBioS Driving Biomedical Projects (DBPs)
DBPs consist of two categories:
- 7 DBPs in progress
- 3 newly added (DBPs 6-8, highlighted in yellow)
- 4 continuing from previous term (DBPs 1-4)
- 1 completed DB (DBP5;shaded in gray).
DBP number and title(a) | Primary Collaborators (PIs) |
TR&D projects |
BTRR Leader(s) and personnel |
How the DBP acts as a driver of test bed? |
External Funding Status (Grant #, title, source and start and end dates if applicable) |
Pubs(b) |
1.Dynamics of Neurotransmitter Transporters: Molecular and Cellular Interactions |
Susan Amara, NIH Gonzalo Torres, U of Florida |
1, 2, 3,4 |
Bahar Cheng, Liu, Faeder, Sejnowski, Bartol |
Site-directed mutagenesis, sulfhydryl modification, and chemical cross-linking approaches and biochemical and elctro-physiological analyses of EAAT3 provide data for building, teseting and refining computational models for anion channeling. Experiments with cell permeable peptide fragments and DAT mutagenesis, in brain synaptosomes and in vivo drive the computational modeling of DAT - G protein βγ complex.
Data on PKA- and RhoA-dependent signaling events and TAAR1 activation will drive the development and benchmarking of BioNetGen model for identifying pathways/targets that involved in AMPH action on DAT. |
1ZIAMH002946-03,Structure, function and pharmacology of neurotransmitter reuptake systems (Amara) 7R01 DA038598 Regulation of dopamine transporter function by G protein β−γ subunits (Torres); 9/15/14 - 6/30/19 |
12, 15 |
2.Regulation and binding to PSD- 95 and its relation to AMPA receptor trafficking |
Mary Kennedy, Caltech |
2, 3, 1
|
Sejnowski Bartol, Wetzel, Hood, Bahar, Faeder |
This project will drive development of the spatial extensions to BioNetGen Language (BNGL) for MCell in two ways, by enabling MCell to deal with combinatorial complexity of the multiple interactions of PDZ domain, and by allowing to including trans-synaptic interactions |
5R01MH095095-04, Time resolved assay of synaptic enzyme activity by mass spectrometry; 7/1/11- 4/30/16 (Kennedy) Caltech Institutional Funds (Kennedy) |
1,2,3 |
Alexander Sorkin, |
1, 2, 3, 4 |
Bahar, Faeder Cheng, Liu Sejnowski, Bartol, Murphy |
Super-resolution time-dependent image data on DA neurons (obtained by EM, immunogold labeling and fluorescence microscopy) using transgenic knock-in mouse model (with HA- tagged DAT) will drive the construction of a spatiotemporally realistic model and MCell simulations of DA reuptake and the effects of drugs/psychostimulants and DA reuptake (influx) or reverse transport (efflux) that will be validated against experiments. |
5R01DA014204-17, Regulation of dopamine transporter by trafficking ; 4/1/01-6/30/22 (Sorkin) |
4, 16-18 | |
Christoph Wuelfing, Bristol (UK) Peter Cullen Paul Verkade |
3, 4
|
Murphy Ramanan, Faeder |
The project is essential to the creation of CellOrganizer functions for aligning and comparing spatiotemporal distributions of multiple molecules and creating causal models |
ERC PCIG11-GA-2012-321554, The spatiotemporal organization of T cell signaling as a regulator of T cell function, European Research Council (Wülfing), 8/1/12-7/31/16 102387/Z/13/Z, Characterising the disruption of signalling dynamics at the immune synapse interface of tumour infiltrating lymphocytes, Wellcome Trust (Wülfing), 8/1/14- 9/30/17 201254/Z/16/Z, Ability of Tumour Infiltrating Lymphocytes (TILs) to Mediate Tumour Killing, Wellcome Trust (Wülfing), 5/1/16-4/30/19 GW4 BioMed DTP, Defining Mechanisms of T Cell Suppression in the Tumour Microenvironment, Medical Research Council (Wülfing), 9/1/16-8/31/19 |
5,6,10* | |
5. Functional connectomics |
Clay Reid, Allen Brain Institute |
4 |
Wetzel Hood |
This project has driven the development of our image alignment software to register and analyze image stacks of 10 to 100 terabytes comprising millions of raw images, and successfully resulted in the publication of the largest-to-date (to our knowledge) network of cortical neurons in Nature 2016. |
5R01NS075436-05, Large-scale connectivity and function in a cortical circuit; 6/15/11-4/30/16 (Reid) |
7,11 |
6. Constructing a dynamic, spatial map of transcription and chromatin structure |
Daniel Larson, NIH |
1, 4 |
Kingsford Murphy, Bahar |
The DBP will drive (i) the extension of protein structure and dynamics analysis software such as ProDy to chromo-some- scale modeling using imaging-derived contact maps and (ii) the development of probabilistic spatial models from movies of one or more image fluorescent spots, from which to derive contact maps and other spatial measurements. |
1ZIABC011383-05, Transcription and splicing dynamics in single cells, NCI Div Basic Sci (Larson) |
- |
Kristen Harris, UT Austin |
2, 3 |
Sejnowski, Bartol, Faeder, Wetzel, Hood |
Content-rich data from EM tomography, serial section EM imaging and analysis will be used to construct spatiotemporally realistic models for neuronal dendrites, axons and synapses, and subcellular components such as ER, mitochondria and endosomes and simulate synaptic function and LTP. |
5R01MH104319-02, Synapse growth and elimination in mature CNS (Harris) 9/26/2014 - 6/30/2019 5R01NS074644-22, Distance- dependent structure and function of neuronal dendrites (Harris) 9/30/97-12/31/16 |
1,2,8,9 | |
8. Scalable approaches to modeling using large sets of rules and images |
Peter Sorger, Harvard |
3, 4 |
Faeder, Murphy |
This DBP will drive the development of integrated tools for model development visualization, calibration, and analysis that are much needed for any modeling project, and the improvement of generative modeling tools that capture biophysical relationships independent of the details of image acquisition and can be used in conjunction with model calibration to study mechanism |
U54-HL127365-02: Pharmaco- response signatures and disease mechanism (LINCS); (Sorger) 9/10/14-6/30/20 DARPA W911NF-14-1-0397 Programmatic modeling for reasoning across complex mechanisms (Sorger) |
(a) the row shaded in gray refers to completed DBP
(b) Publication numbers refer to the list below. Ref 7 was published prior to the start date of the award; Ref 10 is in press.
Publications that resulted from the DBPs:
- Bartol TM, Keller DX, Kinney JP, Bajaj CL, Harris KM, Sejnowski TJ, Kennedy MB (2015) Computational reconstitution of spine calcium transients from individual proteins. Front Synaptic Neurosci 7: 17 PMID: 26500546, PMC4595661
-
Bartol TM, Keller DX, Kinney JP, Bajaj CL, Harris KM, Sejnowski TJ, Kennedy MB (2015) Nanoconnectomic upper bound in the variability of synaptic plasticity. Elife 4.pii: e10778 PMID: 26618907, PMC4737657
-
Stefan MI, Bartol TM, Sejnowski TJ, Kennedy MB (2014) Multi-state modeling of biomolecules. PLoS Comp Biol 10(9):e1003844. PMID: 25254957, PMC4201162
- Cheng MH, Block E, Hu F, Cobanoglu MC, Sorkin A, Bahar I (2015) Insights into the modulation of dopamine transporter function by amphetamine, orphenadrine, and cocaine binding Front Neurol 6: 134 PMID: 26106364, PMC4460958
- Roybal KT, Sinai P, Verkade P, Murphy RF, Wuelfing C (2013) The actin-driven spatiotemporal organization of T-cell signaling at the system scale Immunol Rev 256 (1): 133-47 PMID: 24117818.
- Roybal*, K.T.; T. E. Buck*, X. Ruan*, B. H. Cho, D. J. Clark, R. Ambler, H. M. Tunbridge, J. Zhang, P. Verkade, C. Wuelfing, and R. F. Murphy (2016) Computational spatiotemporal analysis identifies WAVE2 and Cofilin as joint regulators of costimulation-mediated T cell actin dynamics. Science Signaling 9(424): rs3 [*co-first authors].
- Bock DD, Lee WC, Kerlin AM, Andermann ML, Hood G, Wetzel AW, Yurgenson S, Soucy ER, Kim HS, Reid RC (2011) Network anatomy in vivo physiology of visual cortical neurons. Nature 471(7337): 177-82 PMID: 21390124, PMC3095821
- Edwards J, Daniel E, Kinney J, Bartol T, Sejnowski T, Johnston D, Harris K, Bajaj C (2014) VolRoverN: enhancing surface and volumetric reconstruction for realistic dynamical simulation of cellular and subcellular function. Neuroinformatics 12(2):277-89 PMID: 24100964, PMC4033674
- Kinney JP, Spacek J, Bartol TM, Bajaj CL, Harris KM, Sejnowski TJ (2013) Extracellular sheets and tunnels modulate glutamate diffusion in hippocampal neuopil. J Comp Neurol 521(2): 448-64 PMID: 22740128, PMC3540825
- Ambler*, JE, X. Ruan*, R. F. Murphy†, and C. Wülfing† (2017) Systems Imaging of the Immune Synapse. Methods in Molecular Biology. 1584: 409-421 [*co-first authors, †co-senior authors].
- Lee, W. A.; Bonin, V.; Reed, M.; Graham, B. J.; Hood, G.; Glattfelder, K.; Reid, R. C (2016) Anatomy and function of an excitatory network in the visual cortex. Nature 532(7599): 370-374 PMID: 27018655, PMC4844839
- Cheng MH, Torres-Salazar D, Gonzalez-Suarez AD, Amara SG, Bahar I. (2017) Substrate transport and anion permeation proceed through distinct pathways in glutamate transporters. Elife. 6. doi: 10.7554/eLife.25850. PubMed PMID: 28569666; PubMed Central PMCID: PMC5472439.
- Clark D, McMillian L, Tan S, Bellomo G, Massoue C, Thompson H, Mykhaylechko L, Alibhai D, Ruan X, Singleton K, Du M Hedges A, Schwartzberg P, Verkade P, Murphy R, Wulfing C. (2019) Transient protein accumulation at the center of the T cell antigen presenting cell interface drives efficient IL-2 secretion. bioRxiv. NIHMSID: NIHMS1034845. doi: https://doi.org/10.1101/296616
- Bromer C, Bartol TM, Bowden JB, Hubbard DD, Hanka DC, Gonzalez PV, Kuwajima M, Mendenhall JM, Parker PH, Abraham WC, Sejnowski TJ, Harris KM. (2018) Long-term potentiation expands information content of hippocampal dentate gyrus synapses. Proc Natl Acad Sci U S A. 115(10): E2410-E2418. doi:10.1073/pnas.1716189115 Epub 2018 Feb 20. PubMed PMID: 29463730; PubMed Central PMCID: PMC5877922.
- Cheng MH, Garcia-Olivares J, Wasserman S, DiPietro J, Bahar I. (2017) Allosteric Modulation of Human Dopamine Transporter Activity under Conditions Promoting its Dimerization. J Biol Chem 292: 12471-12482
- Ma S, Cheng MH, Guthrie DA, Newman AH, Bahar I, Sorkin A. (2017) Targeting of dopamine transporter to filopodia requires an outward-facing conformation of the transporter. Sci Rep 7: 5399
- Kaya C, Cheng MH, Block ER, Bartol TM, Sejnowski TJ, Sorkin A, Faeder JR, Bahar I. (2018) Heterogeneities in Axonal Structure and Transporter Distribution Lower Dopamine Reuptake Efficiency eNeuro 5: ENEURO.0298-17.2017
- Cheng MH, Ponzoni L, Sorkina T, Lee JY, Zhang S, Sorkin A, Bahar I. (2019) Trimerization of Dopamine Transporter Triggered by AIM-100 Binding: Molecular Mechanisms and Effect of Mutations. Neuropharmacology 161:107676 PMID: 31228486 PMCID: 6917874 DOI: 10.1016/j.neuropharm.2019.107676
Latest News
Upcoming Events
No events |
Past Events
No events |
Collaborate with MMBioS
Research Highlights
The Bahar (TR&D1) and Sorkin (DBP3) labs published an article in the Journal of Biological Chemistry, selected as one of JBC's "Editors' Picks. Our results demonstrate a direct coupling between conformational dynamics of DAT, functional activity of the transporter and its oligomerization leading to endocytosis. The high specificity of such coupling for DAT makes the TM4-9 hub a new target for pharmacological modulation of DAT activity and subcellular localization. (Read more)
Differences in the intrinsic spatial dynamics of the chromatin contribute to cell differentiation
Comparison with RNA-seq expression data reveals a strong overlap between highly expressed genes and those distinguished by high mobilities in the present study, in support of the role of the intrinsic spatial dynamics of chromatin as a determinant of cell differentiation. (Read more)
Nanoscale co-organization and coactivation of AMPAR, NMDAR, and mGluR at excitatory synapses
Work by TR&D2 Investigators and collaborators provide insights into the nanometer scale organization of postsynaptic glutamate receptors using a combination of dual-color superresolution imaging, electrophysiology, and computational modeling. (Read more)
Parallel Tempering with Lasso for model reduction in systems biology
TR&D3 Investigators and collaborators develop PTLasso, a Bayesian model reduction approach that combines Parallel Tempering with Lasso regularization, to automatically extract minimal subsets of detailed models that are sufficient to explain experimental data. On both synthetic and real biological data, PTLasso is an effective method to isolate distinct parts of a larger signaling model that are sufficient for specific data. (Read more)
Image-derived models of cell organization changes during differentiation and drug treatments
Our work on modeling PC12 cells undergoing differentiation into neuron-like morphologies (under C&SP11, completed) has been published in Molecular Biology of the Cell. We have also made the large dataset of 3D images collected in that study available through Dryad. (Read more)
Monoamine transporters: structure, intrinsic dynamics and allosteric regulation
T&RD1 investigators Mary Cheng and Ivet Bahar published an invited review article in Nature Structural & Molecular Biology, addressing recent progress in the elucidation of the structural dynamics of MATs and their conformational landscape and transitions, as well as allosteric regulation mechanisms. (Read more)
Trimerization of dopamine transporter triggered by AIM-100 binding
The Bahar (TR&D1) and Sorkin (DBP3) labs explored the trimerization of dopamine transporter (DAT) triggered by a furopyrimidine, AIM-100, using a combination of computational and biochemical methods, and single-molecule live-cell imaging assays. (Read more)
Pre-post synaptic alignment through neuroligin-1 tunes synaptic transmission efficiency
TR&D2 investigators and collaborators describe organizing role of neuroligin-1 to align post-synaptic AMPA Receptors with pre-synaptic release sites into trans-synaptic “nano-columns” to enhance signaling.(Read more)
Inferring the Assembly Network of Influenza Virus
In an article in PLoS Computational Biology, MMBioS TR&D4 members Xiongto Ruan and Bob Murphy collaborated with Seema Lakdawala to address this question of the assembly network of the Influenza virus.(Read more)
Our findings highlight an important mechanism by which proteins genetically implicated in Parkinson’s disease (PD; PINK1) and frontotemporal dementia (FTD; VCP) interact to support the health and maintenance of neuronal arbors.(Read more)
Improved methods for modeling cell shape
In a recent paper in Bioinformatics, Xiongtao Ruan and Bob Murphy of TR&D4 addressed the question of how best to model cell and nuclear shape.(Read more)
New tool to predict pathogenicity of missense variants based on structural dynamics: RHAPSODY
We demonstrated that the analysis of a protein’s intrinsic dynamics can be successfully used to improve the prediction of the effect of point mutations on a protein functionality. This method employs ANM/GNM tools (Read more)
New method for investigating chromatin structural dynamics.
By adapting the Gaussian Network Model (GNM) protein-modeling framework, we were able to model chromatin dynamics using Hi-C data, which led to the identification of novel cross-correlated distal domains (CCDDs) that were found to also be associated with increased gene co-expression. (Read more)
Structural elements coupling anion conductance and substrate transport identified
We identified an intermediate anion channeling state (iChS) during the global transition from the outward facing (OF) to inward facing state (IFS). Our prediction was tested and validated by experimental study conducted in the Amara lab (NIMH). Critical residues and interactions were analyzed by SCAM, electrophysiology and substrate uptake experiments (Read more)
Integrating MMBioS technologies for multiscale discovery
TR&D teams driven by individual DBPs are naturally joining forces, integrating their tools to respond to the needs of the DBP, and creating integrative frameworks for combining structural and kinetic data and computing technologies at multiple scales. (Read more)
Large scale visualization of rule-based models.
Signaling in living cells is mediated through a complex network of chemical interactions. Current predictive models of signal pathways have hundreds of reaction rules that specify chemical interactions, and a comprehensive model of a stem cell or cancer cell would be expected to have many more. Visualizations of rules and their interactions are needed to navigate, organize, communicate and analyze large signaling models. (Read more)
Integration of MCellR into MCell/CellBlender
Using spatial biochemical models of SynGAP/PSD95, MMBioS investigators were able to merge the MCellR code-base with the MCell code-base and validate its utility and correctness of this sophisticated technology now easily accessible through the MCell/CellBlender GUI. (Read more)
Causal relationships of spatial distributions of T cell signaling proteins
The idea is to identify a relationship in which a change in the concentration of one protein in one cell region consistently is associated with a change in the concentration of another protein in the same or a different region. We used the data from our Science Signaling paper reported last year to construct a model for T cells undergoing stimulation by both the T cell receptor and the costimulatory receptor. (Read more...)
BioNetGen modeling helps reveal immune system response decision
To attack or to let be is an important decision that our immune systems must make to protect our bodies from foreign invaders or protect bodily tissues from an immune attack. Using modeling and experiments, we have painted a sharper picture of how T cells make these critical decisions. (Read more)
Tools for determining the spatial relationships between different cell components
An important task for understanding how cells are organized is determining which components have spatial patterns that are related to each other.Read more
Pipeline for creation of spatiotemporal maps
Using a combination of diffeomorphic methods and improved cell segmentation, we developed a CellOrganizer pipeline for use in DPB4 to construct models of the 4D distributions of actin and 8 of its regulators during the response of T cells to antigen presentation. Read more
Multi-scale Hybrid Methodology
The hybrid methodology, coMD, that we have recently developed [1] has been recently extended to construct the energy landscape near the functional states of LeuT (Fig 1) [2]. This is the first energy landscape constructed for this NSS family member. Read more
Insights into the cooperative dynamics of AMPAR
Comparative analysis of AMPAR and NMDAR dynamics reveals striking similarities, opening the way to designing new modulators of allosteric interactions. Read more
Improved Sampling of Cell-Scale Models using the WE Strategy
The WE strategy for orchestrating a large set of parallel simulations has now been extended to spatially resolved cell-scale systems. The WESTPA implementation of WE has been used to control MCell simulations, including models built using a BioNetGen-CellOrganizer pipeline for situating complex biochemistry within spatially realistic cell models. Read more
Anatomy and Function of an Excitatory Network in the Visual Cortex
MMBioS researcher Greg Hood’s collaboration with Wei-Chung Allen Lee of Harvard University and R. Clay Reid of the Allen Institute for Brain Science concerning the reconstruction of an excitatory nerve-cell network in the mouse brain cortex at a subcellular level using the AlignTK software has been published in Nature. Read more
Molecular Mechanism of Dopamine Transport by hDAT
Dopamine transporters (DATs) control neurotransmitter dopamine (DA) homeostasis by reuptake of excess DA, assisted by sodium and chloride ions. The recent resolution of DAT structure (dDAT) from Drosophila permits us for the first time to directly view the sequence of events involved in DA reuptake in human DAT (hDAT). Read more
Synaptic Facilitation Revealed
An investigation of several mechanisms of short-term facilitation at the frog neuromuscular junction concludes that the presence of a second class of calcium sensor proteins distinct from synaptotagmin can explain known properties of facilitation. Read more
Sparse Graphical Models of Protein:Protein Interactions
DgSpi is a new method for learning and using graphical models that explicitly represent the amino acid basis for interaction specificity and extend earlier classification-oriented approaches to predict ΔG of binding. Read more
Advancing Parallel Bio-simulations
A new non-Markovian analysis can eliminate bias in estimates of long-timescale behavior, such as the mean first-passage time for the dissociation of methane molecules in explicit solvent. Read more